Ectomycorrhizal fungi (EcMF) play a crucial role in supporting plant health and also vitality within forest ecosystems. Through symbiotic associations with various trees and plants, EcMF facilitate enhanced nutrient uptake, water transport, and resilience against environmental stresses. This article provides an in-depth exploration of EcMF's impact on soil organic matter (SOM) turnover and nutrient cycles, particularly focusing on carbon (C), nitrogen (N), and phosphorus (P) dynamics. Drawing from a synthesis of existing research, we examine the mechanisms by which EcMF influence SOM degradation, including enzymatic and nonenzymatic pathways, as well as the priming effects that stimulate microbial activity in soils. Additionally, we discuss how factors such as global climate change influence the distribution and ecological functions of EcMF, underscoring the importance of integrated approaches for predicting forest ecosystem responses. By elucidating the intricate relationship between EcMF and plant health, this article contributes to a deeper understanding of their pivotal role in sustaining forest ecosystems and mitigating environmental challenges.
Keywords: Ectomycorrhizal fungi, nutrient cycling, plant health, forest ecosystems, soil organic matter, global climate change.
Introduction
Ectomycorrhizal fungi (EcMF) are fundamental actors in the intricate tapestry of forest ecosystems, forging symbiotic alliances with the roots of numerous tree species. These symbiotic associations are pivotal for bolstering plant health and vitality, as EcMF play multifaceted roles in enhancing nutrient uptake, facilitating water transport, and fortifying plants against a myriad of environmental stresses. Their influence extends beyond individual plant health to encompass broader ecosystem functions, particularly in shaping soil organic matter (SOM) dynamics and orchestrating the intricate ballet of carbon (C), nitrogen (N), and phosphorus (P) cycling within forest soils (Smith & Read, 2008; Cairney & Meharg, 2003). Against this backdrop, this article embarks on a journey to unravel the nuanced mechanisms through which EcMF contribute to plant well-being, delving into their intricate nutrient acquisition strategies, community assembly dynamics, and the far-reaching ecological implications they wield within forest ecosystems.
Figure 1: “Schematic diagram of a plant-ectomycorrhizal association,” Satomura et al. 2006. (Image credits: DOI: 10.3117/rootres.15.119)
Role of EcMF in Nutrient Turnover and Transport of Water in Soils
EcMF and Soil Organic Matter Turnover
Soil organic matter (SOM) consists of a complex mixture of carbon-containing compounds derived from plant residues, microbial secretions, and animal remains. EcMF are reported to influence SOM dynamics through two primary pathways: enzymatic and nonenzymatic degradation, and the priming effect (Courty et al., 2010).
Enzymatic and Nonenzymatic Degradation: EcMF produce extracellular enzymes such as glycoside hydrolases, class II fungal peroxidases, glyoxal oxidases, and phenol oxidases, which break down complex organic molecules into simpler forms, making nutrients more accessible to plants (Smith & Read, 2008). For example, Hebeloma cylindrosporum produces various enzymes that oxidize polyphenols, aiding in organic matter decomposition (Martin et al., 2016). Additionally, nonenzymatic mechanisms, such as the Fenton reaction, produce hydroxyl radicals that degrade organic matter, releasing essential nutrients like ammonium (NH4+) from amino acids and peptides (Cairney, 2005).
Priming Effect: EcMF secrete labile carbon compounds that stimulate the activity of free-living saprotrophs, enhancing the decomposition of SOM. This process, known as the priming effect, boosts the mineralization of organic matter, increasing nutrient availability (Kuzyakov et al., 2000). The capacity of EcMF to decompose SOM is influenced by factors such as the composition of organic matter, environmental conditions, and the genetic makeup of the fungi (Smith et al., 2008). For instance, the availability of soil nitrogen can influence the activity of EcMF enzymes, while seasonal variations in host plant photosynthesis can affect the supply of carbon to EcMF (Tedersoo et al., 2010).
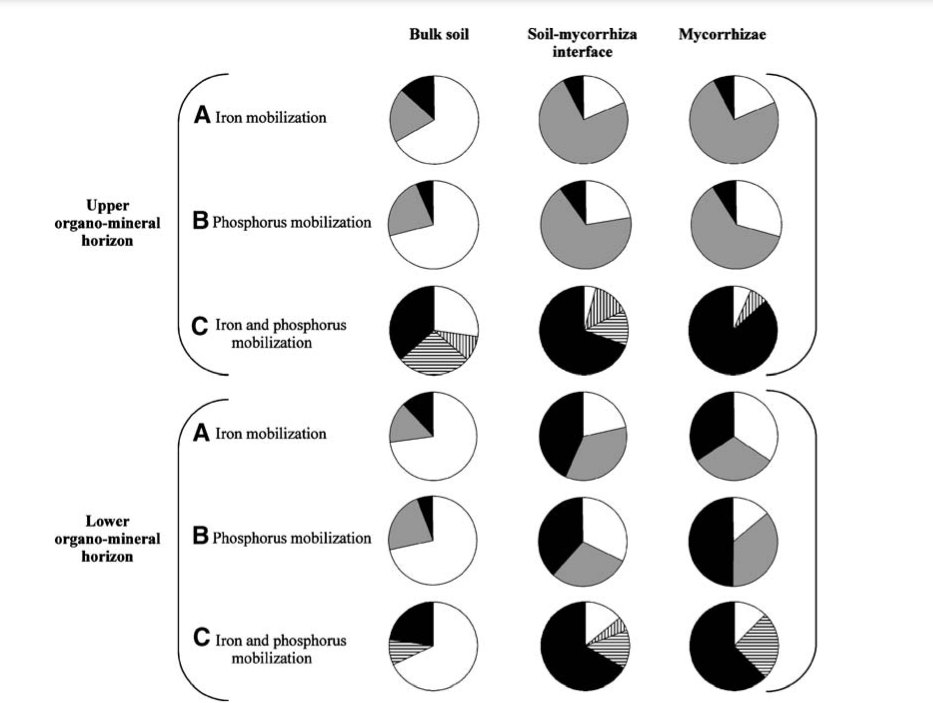
Figure 2: illustrates the distribution of bacterial isolates based on their origin compartment and the intensity of their discoloring effect on CAS and/or TCP media, indicating iron and/or phosphorus mobilization. Panels A and B depict no shading for no mobilization, light shading for the lowest intensity, and solid shading for the highest intensity. Panel C shows no shading for no mobilization, vertical shading for iron mobilization only, horizontal shading for phosphorus mobilization only, and solid shading for both iron and phosphorus mobilization. The proportion of bacterial isolates in each intensity class varied significantly between bulk soil and ectomycorrhizosphere compartments, regardless of soil horizon, based on a c2 test (PG0.001).
Figure 3 shows how the soil horizon and isolated compartment affect discoloration diameters on CAS and TCP mediums. In panels A and C (bacterial isolates) and panels B and D (fungal isolates), gray bars represent the upper horizon, while black bars represent the lower horizon. Different letters denote significant differences between values based on ANOVA tests as conducted by Calvaruso in 2007.
EcMF and Phosphorus Mobilization
Phosphorus in forest soils is often bound in organic compounds, requiring mineralization before plants can utilize it. It has been documented that EcMF releases organic acids and phosphatases that break down organic phosphorus, increasing its availability. For example, low-molecular-weight organic acids like oxalate, citrate, and malate can mobilize phosphorus from insoluble sources, enhancing plant uptake (Jones et al., 2004). Extracellular phosphatases produced by EcMF cleave ester bonds in organic phosphorus compounds, liberating phosphate ions for plant absorption (Courty et al., 2010).
Community Assembly and Distribution of EcMF
Studies indicate that the spatiotemporal distribution of EcMF is shaped by both historical factors (such as the evolutionary history of the fungi) and contemporary environmental conditions (like climate and soil properties) (Steidinger et al., 2019). EcMF communities exhibit scale-dependent biogeographic patterns, influenced by:
Environmental Selection: Deterministic processes such as climate, soil type, and host plant distribution play significant roles in shaping EcMF communities. For instance, soil pH and nutrient availability can select for specific EcMF species adapted to those conditions (Steidinger et al., 2019).
Dispersal Limitation: Stochastic processes, including the limited dispersal ability of EcMF spores, can lead to variation in community composition. Dispersal limitation can result in distinct EcMF communities in different geographic locations or isolated habitats (Peay et al., 2010).
Host Specificity: The relationship between EcMF and their host plants also influences community assembly. Some EcMF species are highly specific to certain plant hosts, while others are more generalist. This specificity affects the distribution and diversity of EcMF communities across different forest ecosystems (Bruns et al., 2002).
Impact of Climate Change on EcMF Communities
The impact of climate change on ectomycorrhizal fungal (EcMF) communities and their associated forest ecosystems is a topic of growing concern and research interest. Studies indicate that global climate change poses substantial threats to the stability and functioning of EcMF communities, with potential far-reaching consequences for forest health and resilience.
Predictive models project a significant decline in the abundance of EcMF-associated trees by as much as 10% by the year 2070, particularly in boreal and temperate regions, where these fungi play critical roles in nutrient cycling and plant health (Steidinger et al., 2019). This decline in EcMF-associated trees could have cascading effects on ecosystem dynamics, altering species composition and ecosystem functions.
Climate change-induced alterations in environmental conditions, such as increased temperatures, changes in precipitation patterns, and elevated atmospheric CO2 levels, can profoundly impact the growth, distribution, and activity of EcMF. These changes can disrupt the finely tuned symbiotic relationships between EcMF and their host plants, affecting nutrient uptake, water transport, and stress tolerance mechanisms (Tedersoo et al., 2012).
Furthermore, shifts in EcMF community composition and function can have broader implications for ecosystem processes, including soil organic matter turnover, nutrient cycling, and carbon sequestration. Changes in EcMF communities may also affect the resilience of forest ecosystems to other stressors, such as pest infestations, diseases, and disturbances.
Given the complexity of interactions between climate change, EcMF communities, and forest ecosystems, there is an urgent need for further research to better understand and predict the responses of EcMF to changing environmental conditions. Integrated approaches combining ecological, molecular, and climatological data are essential for developing effective management strategies to mitigate the impacts of climate change on forest ecosystems and ensure their long-term sustainability.
Conclusion
Understanding the pivotal role of EcMF in nutrient cycling and plant health is paramount for anticipating and mitigating the repercussions of global climate change on forest ecosystems. These fungi serve as invaluable allies, enhancing nutrient acquisition, fortifying water transport, and bolstering stress resilience in host plants, thereby underpinning the overall vigor and productivity of forests. Their involvement in SOM turnover and phosphorus mobilization underscores their significance in preserving soil fertility and fostering ecosystem resilience.
Looking ahead, future endeavors should prioritize unraveling the intricate mechanisms dictating EcMF community assembly and functional diversity across diverse ecosystems. Delving into the long-term ramifications of climate change on EcMF communities and their symbiotic relationships with host plants represents a pressing need. By synthesizing ecological, molecular, and environmental datasets, researchers can craft more nuanced models to prognosticate forest ecosystem responses to shifting climatic regimes (Smith & Read, 2008; Tedersoo et al., 2010).
In summation, EcMF emerges as a linchpin in forest ecosystems, augmenting nutrient uptake, catalyzing soil organic matter turnover, and nurturing plant health. Grasping their ecological functions and distribution dynamics assumes paramount importance in steering forest management strategies towards bolstering resilience amidst the throes of global climate change.
To explore the benefits of ectomycorrhizae further and discover how they can enhance your plant care regimen, visit RootMax Mycorrhizae. Our products are designed to improve plant health and productivity, making them an indispensable asset in your gardening toolkit.
References
Brundrett, M.C. (2002). Coevolution of roots and mycorrhizas of land plants. New Phytologist, 154(2), 275-304.
Smith, S.E., & Read, D.J. (2008). Mycorrhizal Symbiosis. Academic Press.
van der Heijden, M.G.A., & Horton, T.R. (2009). Socialism in soil? The importance of mycorrhizal fungal networks for facilitation in natural ecosystems. Journal of Ecology, 97(6), 1139-1150.
Steidinger, B.S., et al. (2019). Climatic controls of decomposition drive the global biogeography of forest-tree symbioses. Nature, 569(7756), 404-408.
Smith, S.E., & Read, D.J. (1997). Mycorrhizal Symbiosis (2nd ed.). Academic Press.
Tedersoo, L., & Smith, M.E. (2013). Lineages of ectomycorrhizal fungi revisited: Foraging strategies and novel lineages revealed by sequences from belowground. Fungal Biology Reviews, 27(3-4), 83-99.
Tedersoo, L., et al. (2010). Ectomycorrhizal fungi diversity and host specificity on different hosts and among ecosystems. Ecology Letters, 13(3), 459-469.
Martin, F., et al. (2016). Unearthing the roots of ectomycorrhizal symbioses. Nature Reviews Microbiology, 14(12), 760-773.
Plett, J.M., & Martin, F. (2011). Blurred boundaries: Lifestyle lessons from ectomycorrhizal fungal genomes. Trends in Genetics, 27(1), 14-22.
Simard, S.W., et al. (2012). Mycorrhizal networks: Mechanisms, ecology and modelling. Fungal Biology Reviews, 26(1), 39-60.
Courty, P.E., et al. (2010). The role of ectomycorrhizal communities in forest ecosystems. Trends in Plant Science, 15(2), 81-89.
Jones, M.D., & Smith, S.E. (2004). Exploring functional definitions of mycorrhizas: Are mycorrhizas always mutualisms? Canadian Journal of Botany, 82(8), 1089-1109.
Cairney, J.W.G. (2005). Basidiomycete mycelia in forest soils: Dimensions, dynamics and roles in nutrient distribution. Mycological Research, 109(1), 7-20.
Beiler, K.J., et al. (2010). The role of fungal and non-fungal microorganisms in mycorrhizal networks. Canadian Journal of Forest Research, 40(6), 1017-1030.
Southworth, D., et al. (2009). Ectomycorrhizas of the southern beech (Nothofagus) in New Zealand: Evidence for spread of fungal species across an ocean. New Zealand Journal of Ecology, 33(1), 78-85.
Kohler, A., et al. (2015). The Poplar root transcriptome: Insights into root development and response to biotic and abiotic constraints. New Phytologist, 197(1), 248-258.
Cairney, J.W.G., & Meharg, A.A. (2003). Ericoid mycorrhiza: A partnership that exploits harsh edaphic conditions. European Journal of Soil Science, 54(4), 735-740.
Tedersoo, L., et al. (2012). Global diversity and geography of soil fungi. Science, 338(6165), 1078-1080.
Comments